Development of digital bathymetric models from hydroacoustic and photogrammetric data
Geoinformatic techniques to combine bathymetric data for shallow and ultra-shallow waters
•
Unmanned vehicles, both surface and airborne, are increasingly being used for hydrographic surveying. While the technology of data acquisition using these platforms is generally known, the fusion of this data is still the subject of much research, and the methods applied often depend on the sensors used and the properties of the survey area. This study presents a method for fusing data acquired using unmanned surface vehicle (USV) and unmanned aerial vehicle (UAV) measurement platforms to develop a digital bathymetric model. The case analysed concerns shallow water and ultra-shallow water up to the boundary with the shoreline.
Approaches for combining bathymetric data
Various methods are currently used to acquire bathymetric data, including hydrographic methods using hydroacoustic sensors at the water level or below, and remote sensing methods from an aerial platform or satellite. Traditional hydrographic methods include a wide range of survey techniques, ranging from survey poles through single beam echosounders (SBES) to advanced hydroacoustic techniques such as multibeam echosounders (MBES). Remote sensing methods involve the processing of multispectral aerial and satellite images and bathymetric Lidar (Light Detection and Ranging) measurements. It is worth noting that USVs are increasingly being used for many surveying techniques, with the key advantage that they reduce the workload for operators and make it possible to carry out measurements in shallow and restricted waters. UAVs, on the other hand, can be used both as a photogrammetric as well as a Lidar platform. There are also hybrid solutions that enable the acquisition of bathymetry using an echosounder towed by a low-flying UAV or an integrated ground penetrating radar (GPR). Many solutions for hydrographic surveys using USV-based platforms are also increasingly used.
Each of these methods has its limitations and works best in different conditions and types of water bodies. In the case of hydroacoustic surveys, the main constraint is the depth of the water, to allow safe passage of the hydrographic unit, while in the case of remote sensing the parameters of the water and the water body are the main concern, such as water transparency, water depth or bottom type. For this reason, data from different sources is often combined in a fusion process to produce an integrated bathymetric model covering the entire survey area.
Two special areas when it comes to the use of photogrammetry and remote sensing are shallow and ultra-shallow waters, which are usually inaccessible to hydrographic survey units. For effective measurement in these waters, hydrographic and photogrammetric methods must be combined. Unfortunately, different datasets acquired with different sensors have different ranges and structures, related to the density of the points, their quantity or spatial distribution and the measurement errors. The proper integration of data therefore requires the development of dedicated techniques.
Data acquisition in shallow water
This case concerns data acquired by UAV and USV. The study area is part of Lake Dabie in Poland, and includes a small bay with a beach. The area of the investigated region is 2.71ha and the bottom of the water area is mostly flat, with more depression at the entrance to the bay. The average depth of the studied area is about 1m and the maximum depth is 3.95m. Photogrammetric data was acquired with a DJI Phantom Pro surveying platform, using a classical approach with ground control points (GCPs) established on the lake bottom. During image processing, datasets with a density of up to 300 points per square metre with a scattered distribution were obtained. The advantage of this method was the possibility to acquire bathymetric data from the shoreline, through the ultra-shallow to the shallow water. The ultra-shallow water zone is particularly important because, when creating a digital bathymetric model of this area, the lack of bathymetric data cannot always be compensated for using interpolation methods. Previous studies have indicated that doing this results in a much higher compilation error.
Unfortunately, due to poor water transparency, it was effectively possible to acquire data to a depth of 1.3m by using photogrammetric methods. It should be noted here that, for inland waters, depth measurements are in many cases only possible to a depth of 1.6–2m, even using Lidar bathymetric sensors. A natural complement to bathymetric data for greater depths is data acquired by hydroacoustic methods. For this purpose, a USV with SBES sensor was used (see Figure 1).
The survey was performed on planned profiles at intervals of 10m. In the profile, data was acquired approximately 0.3–0.4m apart. The acquired data was processed to improve the quality by analysing individual echograms and selected depths. The advantage of this type of survey is that it is possible to obtain accurate correction data in a single profile and measurements at greater depths. On the other hand, the disadvantage is the inability to survey ultra-shallow depths or areas with navigational hazards (submerged vegetation, branches of submerged trees, fishing nets, etc.). The survey area together with the measurement platforms is illustrated in Figure 2.
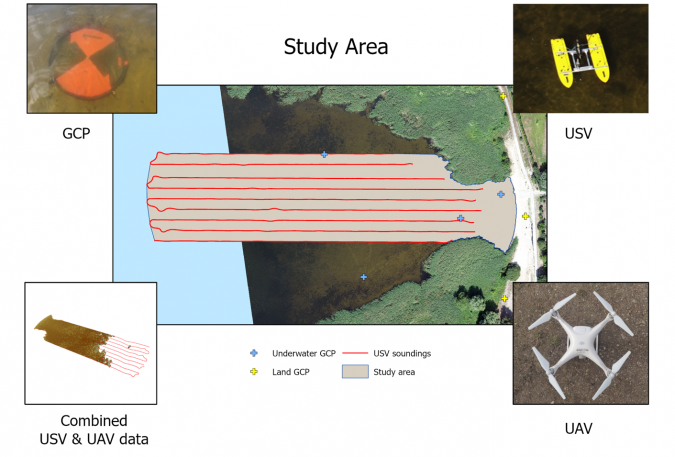
Geoprocessing method
The data fusion approach uses geoinformatic data processing methods and was developed based on the research methodology described in the article listed in the references. The main element of this process is the concept of a bathymetric reference surface on the basis of which a selection is made of post-processed depth data acquired from UAVs. An uncertainty of 25cm was taken as the threshold for acceptable deviations from the bathymetric reference surface. This means that all points within this deviation tolerance were retained. The final processing of the data was preceded by tests to select the appropriate data filter mask, point cloud type and interpolation method. A generalized diagram of the geoprocessing with examples of data is shown in Figure 3.

As the acquisition and processing of the SBES sensor data took place in accordance with the hydrographic methodology used, more attention was paid to the UAV data. During the experiment, photogrammetric data was processed using the traditional method, but with a GCP network established underwater. This approach does not take into account refraction of the electromagnetic wave in water, unlike most of the methods that use passive remote sensing. Additionally, two types of UAV point cloud were analysed. The first was a full cloud, including all point classes, while the second was a ground class cloud. The universal mask for filtering the points was developed on the basis of deviations from the bathymetric reference surface with respect to maximum and minimum values. In the final step, a qualitative and quantitative analysis of the different interpolation methods by which a digital bathymetric model can be developed was carried out.
The analysis indicates that the best results for developing the final product were obtained using a UAV point cloud developed from a ground class cloud. Unfortunately, not all interpolation methods performed well on the data structure consisting of USV profiles and a UAV cloud, such as the Radial Basis Function (RBF) method. The adopted digital bathymetric model (DBM) data processing can be developed using methods such as triangulation, nearest neighbour, kriging or inverse distance to a power, which gives comparable results in terms of accuracy of the reconstructed surface. The developed hybrid bathymetric model is illustrated in Figure 4.
Conclusions
The experiment shows that it is possible to develop a data fusion method and a final bathymetric model based on the proposed geoinformatics data processing for shallow and ultra-shallow water areas. This includes UAV point cloud generation, point filtering using masks and the use of spatial interpolation methods. The essential elements of the proposed method include the acquisition of bathymetric data using a classical photogrammetric approach; in other words, the use of an underwater GCP network, the application of a ground class UAV point cloud and the use of selected interpolation methods. An additional important element of the method is the processing of UAV data based on a bathymetric reference surface. The proposed method certainly simplifies the development of bathymetric data by not taking refraction into account, but its limitations must also be considered. These include an applicability to small areas and the necessity of establishing an underwater photogrammetric network. As photogrammetric methods are formally one of the sources of bathymetric data acquisition, any research that assesses the practical applicability of this type of data and its processing methods is valuable.
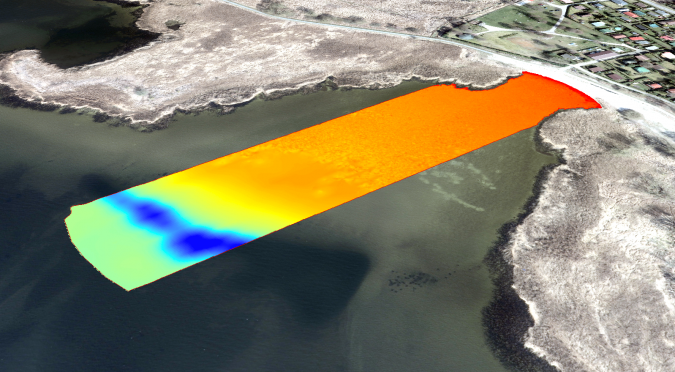
References
Lubczonek, J.; Kazimierski, W.; Zaniewicz, G.; Lacka, M. Methodology for Combining Data Acquired by Unmanned Surface and Aerial Vehicles to Create Digital Bathymetric Models in Shallow and Ultra-Shallow Waters. Remote Sens. 2022, 14, 105. https://doi.org/10.3390/rs14010105
Lubczonek, J.; Wlodarczyk-Sielicka, M.; Lacka, M.; Zaniewicz, G. Methodology for Developing a Combined Bathymetric and Topographic Surface Model Using Interpolation and Geodata Reduction Techniques. Remote Sens. 2021, 13, 4427. https://doi.org/10.3390/rs13214427
Manual on Hydrography. International Hydrographic Organization., IHO C-13. 2011
https://www.hydro-international.com/content/article/development-of-digital-bathymetric-models-from-hydroacoustic-and-photogrammetric-data?gclid=CjwKCAjw-b-kBhB-EiwA4fvKrCgZNK6mBV9CblE8-slbay8zNlgFCcK-BQcPnYM7Qi-agqH57aZDHBoCbDAQAvD_BwE
Comments
Post a Comment